Introduction
Porphyry copper deposits are one of the most important copper exploration targets. However, given the declining discovery rates, innovation is necessary to deliver new insights for exploration decision making, including drill targeting. Ambient Noise Tomography (ANT) has not been used systematically in porphyry copper exploration. Nevertheless, the 3D imaging aspect of the method, coupled with the relative ease with which data can be collected, has resulted in growing interest in the technique for this purpose.
This article provides an overview of the possible velocity response that could be expected from porphyry copper deposits and unpacks the potential value of the ANT method for porphyry exploration. This discussion is presented in light of a recent ANT seismic velocity model released by Fleet Space Technologies and Inflection Resources from the Duck Creek Project, Macquarie Arc, New South Wales (Burrows et al., 2024).
ANT methodology
Ambient Noise Tomography (ANT) is a passive seismic technique that uses Earth’s background noise as the signal for measuring subsurface velocity structure. An array of geophones records the arrival of low frequency surface waves created by natural and anthropogenic seismic sources. This technique is used to construct estimates of seismic Green’s functions (Bensen et al., 2007) between station pairs such that every receiver is turned into a virtual active source that provides information about the subsurface. Inversion of the data can then be applied to construct a full 3D image of the seismic shear-wave velocity (Vs) structure at depth.
Fleet Space Technologies commercialized ANT survey capabilities as part of their end-to-end exploration solution, ExoSphere, which is notably different from the industry norms in two ways (Olivier et al., 2022) (Fig. 1):
[1] Bespoke geophones (Geodes) that provide increased sensitivity to low frequency signals used in the ANT process.
[2] Connecting the Geodes to a satellite network to provide monitoring and rapid processing of uploaded data on a cloud platform.

Porphyry copper deposit geology and possible seismic velocity response
Porphyry copper deposits and related mineral systems are relatively well understood, and the following summary is based on major overview papers (Sillitoe and Perello, 2005; Sillitoe, 2010). Porphyry copper deposits form above subduction zones related to oxidised, hydrous, calc-alkaline magmas in continental and island arcs, typically in a post-collisional tectonic phase (Richards, 2009). The classic porphyry copper deposit model shows the causative porphyry intrusive bodies emanating upwards from a stalled plutonic region at depth (Fig. 2). Geometrically, deposits typically form around or adjacent to steeply-dipping, or formerly steeply-dipping, porphyry intrusions or stocks above source plutons. These features are often pipe-like in geometry, and contain multiple phases of intrusion. Lithologically, these intrusives are often diorite to granodiorite in composition, with variation in hydrous mafic mineral abundance, typically amphibole and biotite, being the main distinguishing feature.
There is a distinct pattern of alteration minerals in porphyry environments from distal propylitic alteration to proximal potassic and quartz-sericite alteration. These alteration zones form as a result of magmatic fluid interaction with reactive country rocks. Copper and accompanying metals such as molybdenum, gold and silver, are typically located in the potassic alteration zone. Chemically reactive rocks such as mafic volcanics, or carbonates can promote precipitation of copper sulphides from magmatic fluids. Epithermal mineralisation may also be present in the porphyry district, if the uppermost levels of the fluid-magma system is preserved (e.g. Voudouris et al., 2019). High and low sulphidation systems often occur in association with breccia pipes and fault zones and typically are dominated by precious metals. Associated with the epithermal systems are zones of intense clay alteration and also silicification.
Each of the components of the prospect-scale geology of porphyry systems will have different density as a result of variation in mineralogy and structure, for example zones of chlorite- and sericite-rich brecciation and fracturing. This variation in density results in variation in seismic velocity. In principle, these velocity variations can be detected by ANT methods, given an appropriate geophone array density, noise conditions, size of the features and other constraining variables.
Figure 2 gives a schematic diagram of a porphyry system and a hypothetical velocity response that attempts to map velocity onto the various geological attributes. We note that it is possible to model this type of system mathematically. Fleet Space Technologies geophysicists have begun this type of synthetic modelling for iron oxide copper-gold deposits, and further modelling work is underway (Gal et al., 2024).
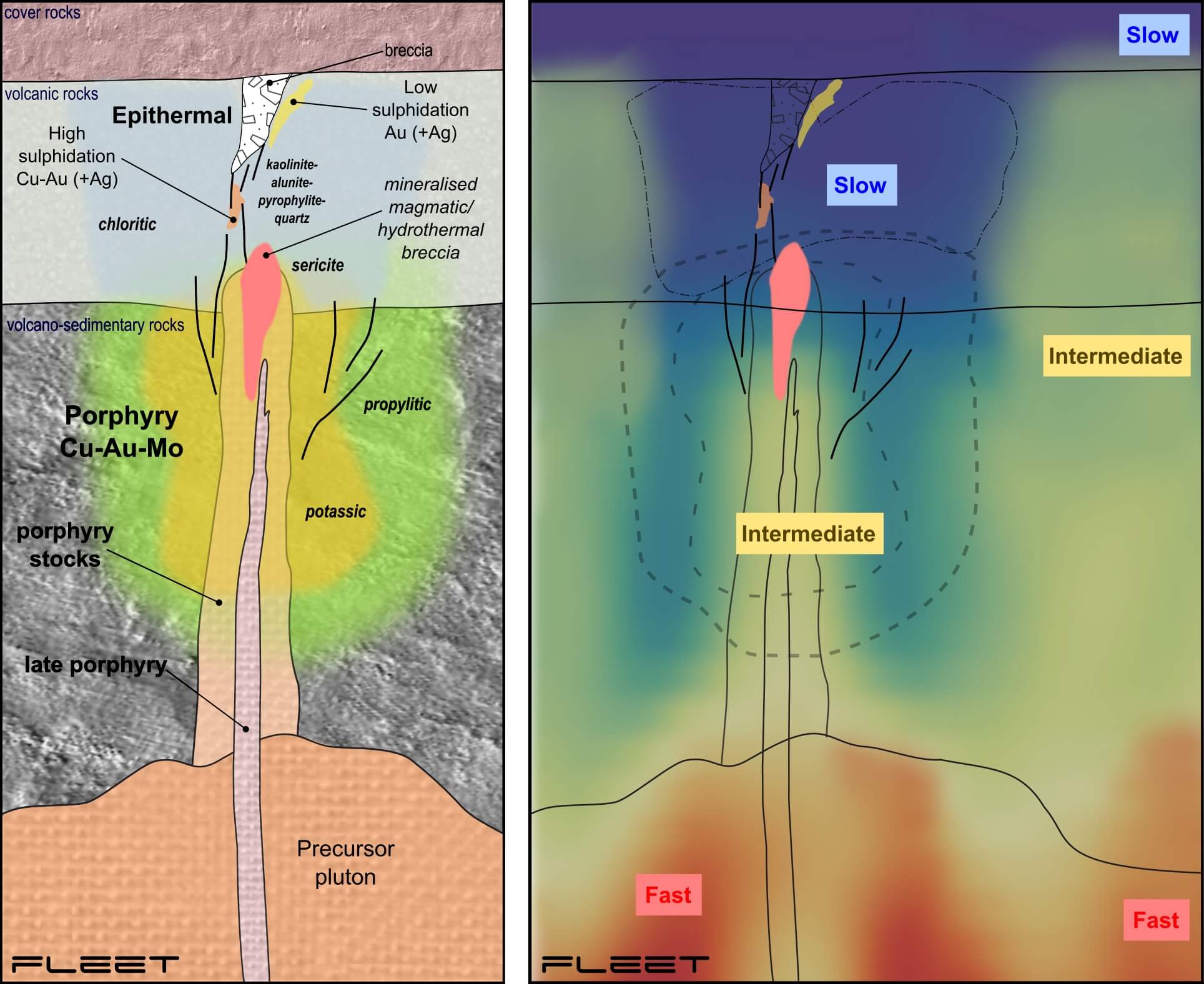
The deeper granitic portions of the porphyry system may have a relatively high seismic velocity. Although velocity generally increases with depth, if these granitic bodies are competent and have intruded into more porous volcano-sedimentary rocks, they will appear as seismically faster than the adjacent rock mass. In detail however, if the host rocks are themselves altered or have structural complications such as faulting, they may be present as low velocities relative to a competent granitic body. It may be difficult to interpret the presence of granitic bodies at depth from the ANT velocity alone, and potential field data will assist in this respect.
A pipe-like intrusive feature extending from a deeper relatively high velocity zone may be an indication of a porphyry intrusion. In principle, such a feature may be relatively seismically fast in comparison to the alteration halo that extends into the surrounding rock mass. Early porphyry intrusions may themselves be highly altered and if so, the ‘core’ of the porphyry system may be seismically slow. Importantly, ANT is effective at imaging sub-vertical structure. This is because ANT measures a range of frequency components, which encode structure at different depths. Vertical structures are sampled by multiple frequencies, which permits robust imaging structures of high inclination compared to seismic reflection techniques that are often limited in this respect.
A substantial, late-stage and unmineralised intrusion may show relatively faster seismic velocity in comparison to the early mineralised and altered intrusions. Likewise, in principle an alteration zone comprising massive K-feldspar in the potassic ‘core’ of the alteration systems may be more dense than an alteration zone dominated by chlorite or sericite. If these features are present, there is a chance that the velocity response of a porphyry alteration system resembles a ‘core’ of higher velocity surrounded by an adjacent zone of relatively lower velocity. If the intrusion system is pipe-like in geometry these velocity features may occur as concentric ‘rings’ of velocity contrast. In general, we expect to see the country rock of the alteration system to present as an intermediate to fast seismic velocity, depending on the rock type. In the example of the Caosiyao molybdenite porphyry, the Precambrian crystalline basement is seismically fast in comparison to the alteration and mineralised zone (Chen et al., 2021).
Importantly, the scale of these features would indeed be greater than the resolution for the particular ANT survey, which in the case of the typical Fleet Space ExoSphere array geometry would mean the feature should be greater than 40m wide for it to be resolvable from the background, noting that the resolution capacity decreases with depth of the feature. Thus it may be that distinct porphyritic intrusions may be too small to be resolved by ANT directly. In this case, an overall velocity low region is the most likely response of a porphyry deposit, since the alteration mineralogy, extensive structuring and fracturing that accompany iteration and mineralisation will reduce the density of the rock mass.
Inflection Resources’ Duck Creek ANT velocity model
Inflection Resources are exploring in the Macquarie Arc, New South Wales and the new survey was conducted at the Duck Creek Project. The Macquarie Arc is highly prospective for porphyry copper systems and is host to the Cadia, Cowal, Northparkes and Boda deposits (Glen et al., 2007; Glen et al., 2012; Kreuzer et al., 2015). The Macquarie Arc lies within the Lachlan Orogen within the Tasmanides of eastern Australia formed as a result of the Paleozoic–Mesozoic paleo-Pacific active margin of east Gondwana (Collins, 2002; Glen et al., 2007; Glen, 2013). The Macquarie Arc is an supra-subduction zone intra-oceanic volcanic arc built on primitive oceanic crust (Glen et al., 2007). The porphyry-related, copper and gold deposits of the region formed during and after amalgamation of the arc with its flanking terranes largely in the Early Silurian. The Duck Creek project in the northern part of the arc lies beneath Permian and Cenozoic sedimentary cover.
Correlation between areas of relatively low velocity and existing drill hole that intersected potassic altered magmatic rocks
The Duck Creek 3D velocity model shows a positive correlation between the intermediate to low velocity zone in the central region of the model with alteration assemblages intersected in drill hole DCKDH002 (Fig. 3). This drill hole intersected variably altered magmatic rocks, including K-feldspar-biotite-magnetite breccia and biotite-magnetite and overprinting sericite-carbonate alteration (Burrows et al. 2024). Pyrite-bearing quartz veins are also present and overprint the potassic alteration assemblages. These assemblages and veins are typical of potassic alteration in porphyry copper environments and give heightened significance to the intermediate to low velocity zones in this area.
In addition, there is a quasi-circular low velocity zone in the north-east of the survey area that has a relatively high velocity central region. The geometry of this relatively high velocity zone is sub-vertical and it appears to be connected to the deeper zones of higher velocity. Inflection resources have highlighted this zone as an area for follow up drilling.

Summary
Porphyry deposits are the main source of copper globally. Their rock type associations, geochemistry, alteration mineralogy and tectonic setting are well understood such that exploration models for porphyry copper deposits have been applied with success (Sillitoe and Perello, 2005; Sillitoe, 2010; Cooke et al., 2014). Nevertheless, the application of ANT is likely to assist in the detection of porphyry alteration systems and porphyry mineralisation, especially in instances where mineralisation is buried beneath sedimentary cover. Each of the zones of porphyry deposits are likely to have variation in seismic velocity due to the variable mineralogy and structure, which may be imaged using ANT methods providing the alteration system is of sufficient size and has sufficient contrast to country rocks.
There are likely two end-member scenarios for using ANT in porphyry exploration. Either the intention is to image the larger scale alteration zones and provide clear vectors towards the source intrusive, or the technique may be aimed at imaging the porphyry stocks themselves. Given there is a larger volume of alteration minerals and the significant velocity contrast between alteration minerals and the ‘background’ country rock, it would seem that ANT is most likely best suited to detecting the alteration footprint rather than individual porphyry intrusions given the relatively small size of typical porphyry stocks.
Inflection Resources partnered with Fleet Space Technologies to undertake camp-scale ANT surveys within their exploration tenements in the Macquarie Arc, New South Wales. The survey results were useful for exploration targeting and have been used as part of their strategy for citing future drill holes in the project area (Inflection Resources, 2024). Some intriguing features in the velocity model may have some parallels in the overall geometry that could be expected from hypothetical velocity responses that could reasonably be expected from a ‘classic’ porphyry alteration system. Further work is required to evaluate the specific causes of the velocity features in the Duck Creek survey, however, the results are intriguing enough to consider that ANT surveys across other prospective porphyry deposit locations may likewise provide companion information to other geophysical data types and, ideally, further drill targets for testing.
Acknowledgements
This article has been prepared by Anthony Reid with input from the geophysicists and geologists of Fleet Space. Fleet Space Technologies acknowledges Inflection Resources' discussions that have furthered our understanding of copper mineral systems in the Macquarie Arc. The interpretations in this article are those of Fleet Space Technologies and are provided as a general guide to the theory of seismic velocity and the effects of alteration on seismic wave speed. Further information on Inflection Resources' exploration can be found on the Inflection Resources website: https://inflectionresources.com/
References
Bensen, G.D., Ritzwoller, M.H., Barmin, M.P., Levshin, A.L., Lin, F., Moschetti, M.P., Shapiro, N.M., and Yang, Y., 2007, Processing seismic ambient noise data to obtain reliable broad-band surface wave dispersion measurements: Geophysical Journal International, v. 169, no. 3, p. 1239–1260.
Burrows, D., Barber, S., and Oyo, A., 2024, Reimaging porphyry copper exploration using Exosphere: Ambient Noise Tomography from the Duck Creek project, Macquarie Arc: Fleet Space Technologies technical case study, available via https://www.fleetspace.com/all-resources, 10 p.
Chen, G., Cheng, Q., Luo, Y., Yang, Y., Xu, H., and Deng, X., 2021, Seismic imaging of the Caosiyao giant porphyry molybdenum deposit using ambient noise tomography: Geophysics, v. 86, no. 6, p. B401–B412.
Collins, W.J., 2002, Nature of extensional accretionary orogens: Tectonics, v. 21, no. 4, p. 1024.
Cooke, D.R., Hollings, P., Wilkinson, J.J., Tosdal, R.M., and Turekian, H., 2014, Geochemistry of porphyry deposits: Treatise on geochemistry, v. 13, p. 357–381.
Gal, M., Oliver, G., Lecocq, T., and Gunner, G., 2024, Assessing the Accuracy and Feasibility of Ambient Noise Tomography for Copper Exploration: Insights from Synthetic Data Generation with Realistic Geological Models: Copernicus Meetings EGU24-13672.
Glen, R.A., 2013, Refining accretionary orogen models for the Tasmanides of eastern Australia: Australian Journal of Earth Sciences, v. 60, no. 3, p. 315–370.
Glen, R.A., Crawford, A.J., and Cooke, D.R., 2007, Tectonic setting of porphyry Cu – Au mineralisation in the Ordovician – Early Silurian Macquarie Arc, Eastern Lachlan Orogen, New South Wales: Australian Journal of Earth Sciences, v. 54, nos. 2-3, p. 465–479.
Glen, R.A., Quinn, C.D., and Cooke, D.R., 2012, The Macquarie Arc, Lachlan Orogen, New South Wales: its evolution, tectonic setting and mineral deposits: Episodes, v. 35, no. 1, p. 177–186.
Inflection Resources, 2024. Inflection Resources Provides Update on Geophysical Surveys – Multiple New Drill Targets Defined on Duck Creek Project. News release, April 8, 2024.
Kreuzer, O.P., Miller, A.V.M., Peters, K.J., Payne, C., Wildman, C., Partington, G.A., Puccioni, E., McMahon, M.E., and Etheridge, M.A., 2015, Comparing prospectivity modelling results and past exploration data: A case study of porphyry Cu–Au mineral systems in the Macquarie Arc, Lachlan Fold Belt, New South Wales: Ore Geology Reviews, v. 71, p. 516–544.
Olivier, G., Borg, B., Trevor, L., Combeau, B., Dales, P., Gordon, J., Chaurasia, H., and Pearson, M., 2022, Fleet’s Geode: A Breakthrough Sensor for Real-Time Ambient Seismic Noise Tomography over DtS-IoT: Sensors , v. 22, no. 21.
Richards, J.P., 2009, Postsubduction porphyry Cu-Au and epithermal Au deposits: Products of remelting of subduction-modified lithosphere: Geology, v. 37, no. 3, p. 247–250.
Sillitoe, H.R., 2012, Copper Provinces, in Hedenquist, J.W., Harris, M., and Camus, F. eds., Geology and Genesis of Major Copper Deposits and Districts of the World: A Tribute to Richard H. Sillitoe: Society of Economic Geologists, p. 1–18.
Sillitoe, R.H., 2010, Porphyry copper systems: Economic geology, v. 105, no. 1, p. 3–41.
Sillitoe, R.H., and Perello, J., 2005, Andean copper province; tectonomagmatic settings, deposit types, metallogeny, exploration, and discovery: Economic Geology 100th anniversary volume, 1905-2005, p. 845–890.
Voudouris, P., Mavrogonatos, C., Spry, P.G., Baker, T., Melfos, V., Klemd, R., Haase, K., Repstock, A., Djiba, A., Bismayer, U., Tarantola, A., Scheffer, C., Moritz, R., Kouzmanov, K., et al., 2019, Porphyry and epithermal deposits in Greece: An overview, new discoveries, and mineralogical constraints on their genesis: Ore Geology Reviews, v. 107, p. 654–691.